Overview
4. Regrowth Simulation
5. Regrowth Regimen
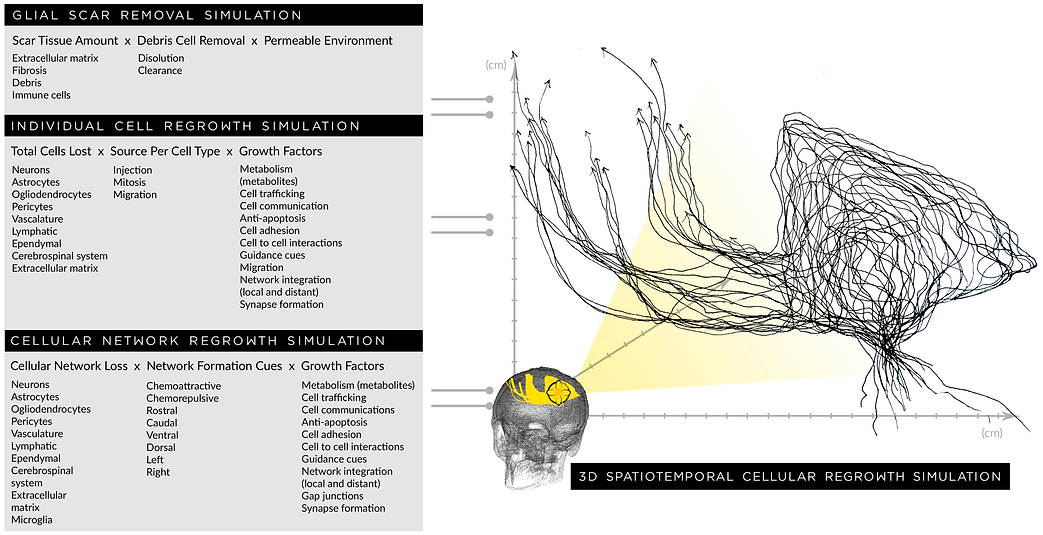
Problem to be Solved by h.o.p.e.
The human central nervous system is composed of a trillion cells. Even a 1% loss of tissue is 10 billion cells extending over collective miles of cellular networks. No research group has the ability – or has even tried – to calculate precise, comprehensive cellular requirements for neurological regrowth on a rodent level, let alone a human scale. The CNS is too complex to be fixed with a single agent, and the daunting complexity of repair must be calculated in terms of what is required to replace and reconnect the patient’s missing neurological tissue. Therefore, we must build the necessary logic engine, or “repair manual,” to match the thousands of treatment points onto the correct scale and scope of neurological damage, to be a repository for our evolving regrowth strategies, and to offer the means to track experiments and learn from them.
Technology to be Orchestrated
Cell Biology
The global scientific community has spent generations assembling a knowledge base of the 30,000 proteins in a single cell and the trillion cells in the CNS. Everything from DNA replication, RNA production of proteins, and the assembly of these proteins into the workhorses of the cell is described in exceptional detail. Institutes such as Roche Scientific and the Ventner Institute have impressively compiled this information into a single chart and mathematically modeled a single cell, respectively. There is an absolute wealth of information on guidance factors (proteins that control cell and cellular processes movement), growth factors (proteins responsible for cell survival and viability), metabolism (the processing of substrates for energy), blood supply (the complex flow of metabolites), cell communication (the regulation of information between cells), network integration (local, regional and distant), gap junctions (open pores between cells critical for cell regulation and communication), synapse formation (the unit of transmission of information between neurons), etc. This scientific exploration of biology produces daily insights and transforms how we understand life year after year.
Computer Modeling
The ability to follow thousands of steps in an organized fashion has increased across multiple fields. Informatics continue to make great strides in developing the methods, algorithms, and tools necessary to unravel the complexities of enormous data. There are rigorous computational methods integrating medical and experimental observations with systems and chemical biology to explain drug effects and to further our understanding of basic biology and human disease. These fields are at the forefront of the use of high-throughput data-capture technologies - such as genome and transcriptome sequencing, metabolomics and proteomics.They use empirical medical observations to study the complex interplay among genetics, the environment, and disease. These technologies improve the modeling of an initial biochemical signal with the progression of a broad spectrum of molecular events. This progress also extends into 1) modeling the multiscale dimensions required in tissue engineering; 2) increasing the power of computing capabilities; and 3) the integration of predictive models among biology, materials science, and physical stimuli on cell phenotype. All of this progress will be used to develop a patient-specific multiscale (both cellular and cell network) model.
Computing power and access to this processing power are also rapidly increasing. Use of the supercomputing grid allows the development of models with high enough complexity in their resolution and in the management of databases to make significant progress toward modeling a single patient.
Modeling extreme complexity can also make use of the experience and expertise offered by other fields. Of particular relevance on the micrometer and angstrom (cellular) scale is industrial engineering, which has expertise and operating procedural models to build computer chips in clean rooms requiring thousands of exacting steps to be perfectly performed in the correct sequence on microscopic scales. On integrating multiple materials, architectural blueprints encapsulate the vast array of different materials and expertise that must be coordinated in the correct sequence and timeline to build towering skyscrapers. On a network scale, energy grids have the software to build, monitor, and manipulate the production and flow of electricity from thousands of energy sources across expansive networks. The scales are enormous, the teams are large, and the budgets are significant. However, the ability to plan and execute thousands and thousands of decision points is not new and has been repeatedly performed, as witnessed by viewing any city skyline lit up at night.
Data Visualization
Informatics is ever improving in terms of the challenge of visualizing data and information. The field is striving to create higher “data-to-ink ratios,” i.e., to layer more data into a figure with finer detail available to the user. Several companies can provide holographic visualization of scientific data with the most up-to-date 3D imaging techniques, or other complex engineering data, to produce digital holographic models for scientific and research display purposes.
Goal of h.o.p.e.
We will build a 3D spatial and temporal regrowth simulation designed to match treatment strategies for unique neurological injuries. We will master the complexity of cell biology by using machine learning, computational biology, and computer modeling in an evolving logic platform. This regrowth simulation will establish a treatment regimen that calculates on a cell-by-cell basis the total amount of therapeutics required.
This means, for the unique injury visualized by the 3D missing tissue hologram, the calculations will begin with
The removal of the glial scar around the injury cavity:
-
How is the patient’s specific amount of glial scar removed (enzymes, small molecules, viral vector)?
-
How is the dissolved scar tissue cleared from the injury site?
-
How is the permeable environment maintained?
Cell replacement of all cell type:
-
What will the new cell sources be (stem cells, mature cells, subventricular zone, mitosis, migration)?
-
What are the cell survival requirements for each cell type (growth factors, metabolism, blood supply, cell trafficking, cell communication, apoptosis, cell adhesion, cell-cell interactions, guidance cues, gap junctions)?
-
What is the sequence and timeline for each cell-type regrowth?
Cellular network regrowth:
-
What are the migration cues and gradients (excitatory, inhibitory, rostral, caudal, ventral, dorsal)?
-
What are network survival requirements for each cell type (growth factors, metabolism, blood supply, cell communication, network integration – local, regional, and distant, gap junctions, synapse formation)?
-
What is the sequence and timeline for each cellular network regrowth?
These survival and functional calculations for every missing neuron, astrocyte, oligodendrocyte, pericyte, vasculature cell, lymphatic cell, ependymal cell, cerebrospinal system cell, extracellular matrix configuration, and microglia cell regarding the absolute number of required growth factors, metabolites, apoptosis cues, cell adhesions, migration guidance cues, nutrient gradients, network integration factors (local, regional, and distant), gap junctions, and synapses will be orchestrated regarding the dosing, gradient, sequence, and duration of treatment. This calculation will be the template for the regrowth regimen design section and will be tested in the lab clinical trial to ensure tissue regrowth would unfold as predicted.
Impact of h.o.p.e.
This will be the first attempt to match the scale required for the regrowth of an individual’s unique neurological injury with all known pro-growth cellular knowledge in a repair strategy built around a complete understanding of all the involved cells, their networks, and functional requirements.
This is a comprehensive strategy to achieve central nervous system organogenesis, one that understands the complexity of the problem and tackles each challenge systematically.
If billions of cells are lost, how do we correctly replace those billions of cells? If a meter-long neural network is gone, how do we regrow that meter-long neural network? How much glial scar is present? How do we exactly bypass the inhibitory glial scar? Which cells need to be repopulated first? Second? Third? How far do all cells need to travel to integrate into their respective functional networks? How long for each axon to reach its target? How much growth factor is needed? Each of these questions, as well as many others, needs to be answered. A cell does not move without a combination of upstream guidance molecules cueing the internal cellular machinery to move. Therefore, if we want a cell to move 500 millimeters and then turn left, we have to provide it with the correct amount of drugs to travel 500 millimeters and turn left. The current state of science does not have the capacity to answer these vital questions and falsely hopes that the cells have enough inherent knowledge to fix themselves. Unfortunately, when cells formed the correct neural networks, we were in utero, about the size of a grape, and our neural networks stretched rather than migrated. For a new neural network to migrate adult distances after an injury is unprecedented, and we must assist the cells in their attempts at regrowth. We must significantly “nurture” our “nature.”
This proposal is predicated on the belief that massive gaps in logic have caused these extreme failures of translational medicine. Easy examples are gathered from the fact that very few neuroscience experiments factor in blood supply into their repair strategies. Without a blood supply, the attrition rate of every stem cell injection is catastrophic. The numbers from previous trials demonstrate injections of millions of stem cells leads to the survival of only thousands, and those surviving were likely just luckily near a blood supply. Additionally, after a brain/spine injury, scar tissue forms around the damaged area, which prevents new cells from migrating to and through the area. The combination of this scar tissue and the lack of blood supply leaves all stem cell trials with trapped cells that quickly die. This proposal’s new level of comprehension will help to create a more sophisticated approach to increase the speed, scale, and success of tissue regrowth.
It is imperative that these vital questions be addressed and orchestrated with the use of current best practices. A logical, organized computer model is vital toward the goals of not wasting time or resources, learning from failures, handling the staggering complexity (billions of cells at the nanometer and molecular level), being the repository of new knowledge, and creating the necessary clinical protocols, which will be used for the patient.